Resonance Structure Generator
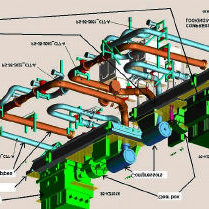
The concept of resonance in chemistry and physics is a fundamental principle that underlies many phenomena, from the behavior of molecules to the properties of solids. At its core, resonance refers to the ability of a system to oscillate or vibrate at specific frequencies, often in response to external stimuli. This concept is crucial in understanding various chemical and physical processes, and its application extends far beyond the confines of these disciplines, influencing fields such as materials science, pharmacology, and even quantum mechanics.
To delve into the intricacies of resonance structure, it’s essential to first grasp the basic principles of molecular structure and how electrons are distributed within molecules. In traditional valence bond theory, molecules are described using Lewis structures, which depict the arrangement of electrons in a molecule. However, for many molecules, a single Lewis structure cannot adequately describe the distribution of electrons or the molecule’s stability. This is where resonance structures come into play.
Resonance structures are a set of Lewis structures that contribute to the overall electronic structure of a molecule. These structures are hypothetical and are used to describe the delocalization of electrons within the molecule. Delocalization is a key concept here, as it refers to the distribution of electrons over multiple atoms or throughout the molecule, rather than being localized between two specific atoms. This delocalization leads to increased stability of the molecule, as it allows the electrons to occupy more favorable energy states.
A classic example of resonance structures can be seen in the benzene molecule (C6H6). Benzene is a planar, ring-shaped molecule composed of six carbon atoms, each bonded to a hydrogen atom. In terms of Lewis structures, benzene can be represented by two main resonance structures, each with alternating double bonds between the carbon atoms. However, neither of these structures alone accurately describes benzene, as the actual molecule does not have distinct single and double bonds between the carbon atoms. Instead, the electrons in the p orbitals of the carbon atoms are delocalized around the ring, resulting in a more stable configuration than any single resonance structure could depict.
The generation of resonance structures involves several steps and considerations. First, one must be able to draw the basic Lewis structure of the molecule, ensuring that the octet rule is satisfied for all atoms (except hydrogen, which requires two electrons). Next, any atoms that can participate in delocalization (typically those with available p orbitals) are identified. The resonance structures are then generated by rearranging the electrons in a way that satisfies the octet rule for all atoms involved in the delocalization, while also ensuring that the total number of electrons remains constant across all structures.
The resonance structures are not equally contributing in all cases; some structures may contribute more to the overall electronic structure of the molecule than others. The contribution of each structure is determined by its stability, with more stable structures contributing more significantly. The stability can be influenced by several factors, including the electronegativity of the atoms involved, the presence of electron-donating or electron-withdrawing groups, and the overall symmetry of the molecule.
In conclusion, resonance structures are a fundamental tool in understanding the electronic distribution within molecules and the stability that arises from electron delocalization. By considering the resonance structures of a molecule, chemists and physicists can gain insights into the chemical properties and reactivity of the molecule, information that is crucial in the design of new materials, drugs, and technologies.
Practical Applications of Resonance Structures
The practical applications of resonance structures are diverse and far-reaching. In materials science, understanding the resonance structures of molecules can help in designing materials with specific properties, such as conductivity or optical properties. In pharmacology, the resonance structures of drug molecules can influence their interaction with biological targets, affecting their efficacy and safety profile. Even in quantum mechanics, resonance plays a critical role, as it underlies many quantum phenomena, including quantum tunneling and resonance in quantum systems.
Future Directions
As our understanding of molecular electronics and quantum computing advances, the concept of resonance structures will continue to play a pivotal role. Research into new materials and molecular devices will rely heavily on the ability to predict and manipulate the electronic properties of molecules, tasks for which understanding resonance structures is essential. Furthermore, advances in computational chemistry will enable more accurate predictions of molecular properties and reactivity, further highlighting the importance of resonance in molecular science.
Decision Framework for Applying Resonance Structures
For researchers and scientists looking to apply the concept of resonance structures to their work, a systematic approach is necessary. This includes:
- Identifying Applicable Systems: Determine if the molecule or system of interest can exhibit delocalization and thus benefit from a resonance structure analysis.
- Drawing Initial Structures: Draw the basic Lewis structures of the molecule, considering all possible arrangements of electrons.
- Generating Resonance Structures: Rearrange electrons to generate all plausible resonance structures, considering rules for delocalization and stability.
- Evaluating Contributions: Assess the relative contribution of each resonance structure to the molecule’s overall electronic structure, based on stability and other factors.
- Interpreting Results: Use the generated resonance structures to predict chemical properties, reactivity, and potential applications of the molecule.
Conceptual Exploration: Beyond Classical Resonance
The concept of resonance extends beyond the classical chemical structures to influence our understanding of quantum systems. In quantum mechanics, resonance refers to the phenomenon where a quantum system oscillates between two or more states. This quantum resonance has implications for quantum computing, quantum communication, and even the understanding of certain types of chemical reactions at the quantum level.
As we delve deeper into the quantum realm, the principles of resonance will continue to evolve, offering new insights into the fundamental nature of matter and energy. The exploration of quantum resonance phenomena will undoubtedly uncover new areas of application, from enhancing the efficiency of quantum devices to understanding complex biological processes at the molecular level.
Technical Breakdown: Delocalization in Solids
Delocalization, a key concept in the discussion of resonance structures, also plays a critical role in the physics of solids. In metals, for example, electrons are delocalized over the entire crystal lattice, leading to high conductivity. This delocalization is a result of the overlap of atomic orbitals, similar to the mechanism in molecular resonance structures, but on a much larger scale.
Understanding delocalization in solids requires a technical breakdown of the band structure of materials. The band structure refers to the range of energy levels that electrons can occupy in a solid. In metals, the valence band and conduction band overlap or are very close, allowing electrons to easily move between them and conduct electricity. This phenomenon is directly related to the concept of resonance and delocalization seen in molecular structures.
FAQ Section
What is the main purpose of using resonance structures in chemistry?
+The main purpose of using resonance structures is to describe the delocalization of electrons in molecules, which cannot be adequately represented by a single Lewis structure. This helps in understanding the stability, reactivity, and properties of the molecule.
How do resonance structures contribute to the stability of a molecule?
+Resonance structures contribute to the stability of a molecule by allowing the delocalization of electrons. This delocalization leads to a lower energy state for the molecule, as the electrons are able to occupy more favorable energy levels. The more resonance structures a molecule has, the more stable it tends to be, as the energy of the molecule is lowered due to the delocalization of electrons.
Can any molecule exhibit resonance?
+No, not all molecules can exhibit resonance. For a molecule to exhibit resonance, it must have electrons that can be delocalized. Typically, this involves the presence of double or triple bonds, lone pairs, or other electrons that can move freely within the molecule. Molecules with only single bonds between atoms (saturated molecules) do not usually exhibit significant resonance.